Max Planck Turbulence Facility
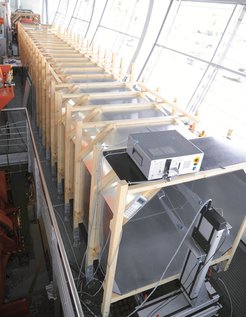
To understand turbulence in a fundamental sense and to make predictions useful in real-world applications, one needs not only to observe turbulence at high Reynolds numbers, but also to realize flows with various spatial and temporal large scale properties. These well defined flows must go hand in hand with precise measurements. In addition one must have the ability to adjust the conditions in various ways, so that dependencies can be uncovered. The facilities at the MPI DS make it possible not only to generate turbulence with the highest Reynolds numbers yet possible under laboratory conditions, but to do so with unprecedented control. To match our achievements in controlling turbulent flows, we have advanced measurement technologies, and have even adapted these to field experiments of natural flows.
The Variable Density Turbulence Tunnel (VDTT) [1] is a recirculating pressurised wind tunnel that consists of two measurement sections with cross-sectional areas of 1.9m2 and lengths of 9m and 7 m. The maximum flow speed is approximately 5.5 m/s. The chief merits of the VDTT are that it produces high Reynolds number flows and stable operating conditions. Furthermore, the Reynolds number is finely adjustable by changing the pressure of the gas in the tunnel, usually sulphur hexafluoride (SF6) up to a pressure of 15 bar. In its current configuration, an active grid consisting of 111 individually controllable flaps creates turbulent wakes of variable size and intensity [2, 3]. This ultimately influences the homogeneity, isotropy, and intensity of the turbulent fluctuations. The active grid allows us to reach Reynolds numbers up to 6000 compared to maximally 1700 when a classical grid of crossed bars is used to inject turbulent kinetic energy. There exists no other wind tunnel facility that can achieve comparable Reynolds numbers. Additionally, the active grid gives us precise control over the turbulence forcing. Recently, a stationary particle-tracking system was implemented and tested successfully. With this, the facility has tripled the Reynolds number of Lagrangian measurements available to international turbulence research. Key elements include a 300W laser illumination, an in-house particle disperser, and a vibration-damped platform holding four state-of-the-art high-speed cameras.
The Prandtl tunnel is an open-circuit wind tunnel dating to the 1930’s. The wind tunnel is 11 meter long with a measurement section of 1.2m x 1.5m and maximum wind speed of 12 m/s. It can be equipped with hotwire anemometers and thanks to the large measurement section it is suitable for a large variety of experiments. A version of the active grid implemented in the VDTT is also available for flexible turbulence generation.
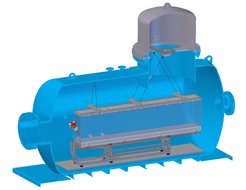
The High Pressure Convection Facility (HPCF) [4] utilizes a generalpurpose pressure vessel called the “U-Boot”, which is 5.3m long and has a diameter of 2.5m and can be filled with SF6 up to a pressure of 19 bar. We precisely control both the temperature and the pressure in the vessel. Within the vessel is a rectangular Rayleigh-Bénard (RB) experiment of large aspect ratio (height 0.7 m, length 3.5m and width 0.35 m) that can reach Rayleigh numbers as high as 5×1013. With its transparent sidewalls, the experiment allows for optical velocity measurements at unprecedented large Rayleigh numbers.
The “Cigar” is a general-purpose pressure vessel with a length of 4m and an inner diameter of 1.5 m. It can be filled with SF6 up to a pressure of 19 bar to perform smaller convection or turbulence experiments or to test equipment for the other pressurized facilities.
Two von Kármán mixers generate high Reynolds number turbulent water flows between two counter-rotating baffled disks. Because the average displacement of fluid particles near the middle of the mixers is close to zero, their motions can be followed for a long time. The mixers are about a half-meter in diameter, and Rλ can be as high as 1200. Large glass windows provide optical access for imaging techniques. The apparatus can be pumped down to reduced-pressure for the study of bubble dynamics. A frequency doubled high-power (50 W), high-repetition-rate Nd:YAG laser is devoted to measurements in this apparatus.
Theoretical knowledge is most developed for turbulence that is stationary and isotropic. But real flows are neither. Three novel apparatuses [5, 6], make it possible for the first time to control the degree to which a turbulent flow is anisotropic both, in gases and in water. We produce cloud-like conditions in one soccer ball.
The facilities make use of state-of-the-art three-dimensional Lagrangian particle tracking (LPT) technologies that we have developed in-house. The technology relies on multiple ultra high-speed cameras viewing the same particles from different angles, with megapixel resolution and kilohertz frame rates. Recently we have developed a techniques that enables us to measure the 3D vorticity in water flow. We also employ a Dantec hot-wire system in conjunction with nanofabricated hot-wires from Princeton University, a LaVision tomographic particle image velocimetry system, and a TSI laser Doppler velocimetry and particle sizing system. All of this equipment is compatible with pressures up to 15 bar. Some of these techniques require substantial light, which is typically produced by Nd:YAG lasers or argon-ion lasers. The systems produce data at rates that necessitate high-performance computing and storage clusters.
The Max Planck Turbulence Facilities (MPTF) are open to visiting researchers. For example it has been used in the European High- Performance Infrastructures in Turbulence (EuHIT) project. It aims to integrate cutting-edge European facilities for turbulence research across national boundaries [7, 8].
[1] E. Bodenschatz, G. P. Bewley, H. Nobach, M. Sinhuber, H. Xu, Rev. Sci. Instrum. 85, 093908 (2014)
[2] K. P. Griffin, N. J. Wei, E. Bodenschatz, G. P. Bewley, Exp. Fluids 60, 55 (2019)
[3] C. Küchler, G. Bewley, E. Bodenschatz, J. Stat. Phys. 175, 617 (2019)
[4] G. Ahlers, D. Fünfschilling, E. Bodenschatz, New J. Phys. 11, 123001 (2009)
[5] K. Chang, G. P. Bewley, E. Bodenschatz, J. Fluid Mech. 692, 464-481 (2012)
[6] R. Zimmermann, H. Xu, Y. Gasteuil, M. Bourgoin, R. Volk, J.-F. Pinton, E. Bodenschatz, Rev. Sci. Instrum. 81, 055112 (2010)
[7] http://www.euhit.org
[8] http://www.euhit.org/media/EuHIT_EN.480p.mp4