Biological data transmission
Energy efficient hearing by enforced nanoscale calcium signaling
Taking the short route: nanoscale calcium signaling at hair cell synapses
Sensory and nerve cells forward information through specialized cell junctions called synapses. Here, the information is transmitted by tiny packages (vesicles) containing neurotransmitter molecules that are released by one of the cells and that can be detected by the neighboring cell. The "sending" cell conveys the order to release the neurotransmitters by means of calcium ions. In its cell membrane, there are molecular "pores"—so-called ion channels—that sense the excitation of the cell and allow calcium ions to enter when a certain threshold is reached. In hair cells, these pores are the key translation device between the acoustic signals and the nerve impulses that are sent to the brain.
In order to fulfill its signal transmission mission, the calcium ions must quickly reach a sensor protein on the vesicles. The Max Planck researchers have calculated that this molecular sensor is located less than 20 nanometers (about 200 atomic diameters) from the ions entry point into the cell. Because they are randomly hit by the surrounding water molecules, it is physically inevitable that many of the entering ions move into wrong directions or overshoot the target location. Without special precautions these misguided ions could trigger the release of neurotransmitters outside the contact points—which would be wasteful as they would go undetected outside the synapse. Such a misplaced release would be a significant waste of energy as the vesicles and messengers must be expensively recycled after each release.
With their new results, the Göttingen researchers show that the hair cells in the inner ear use special calcium buffering proteins to capture ions that go astray. By means of a high concentration of three different calcium buffering proteins, the cells avoid the waste of energy.
Combining experimental and mathematical cell research
In order to figure out what exactly happens in the hair cell of the ear during the release of neurotransmitters, the Göttingen research team led by professors Tobias Moser and Fred Wolf has combined genetic, cell biological, mathematical and computational methods.
The work was made possible by a triple-knockout mouse-developed by Professor Beat Schwaller-in which all essential calcium buffering proteins of the hair cells are genetically eliminated. Using this mouse, Tina Pangrsic and Nicola Strenzke at the University Medical Center Göttingen could evaluate for the first time changes in the transmitter release and the neural coding in the absence of calcium buffer. "By measuring the transmitter release of the cell, we noted that a lot more vesicles are released in the triple-knockout mouse," says Tina Pangrsic. The researchers demonstrated this by means of a technique developed by Erwin Neher in Göttingen, which allows precise measurements of the cell surface that increases by a tiny area every time a vesicle is released. "However, in the auditory nerve that receives the hair cells’ transmitters via synapses, this additional release is not detectable, the cells act completely normal," Nicola Strenzke adds. "Hence, an unnecessarily large machinery is set in motion inside the hair cell, and transmitters are released outside the synapse where they cannot fulfill their job."
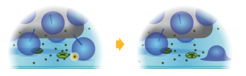
Calcium takes the local route. Calcium ions need to travel only a one-molecule-scale distance to reach neurotransmitter containing vesicles and trigger release in auditory hair cells.
The two Max Planck researchers Mantas Gabrielaitis and professor Fred Wolf developed a computer model that calculates the movements of calcium ions and the role of the calcium-binding proteins. "We wanted to know how the hair cells accomplish to concentrate the neurotransmitter release “command” at the right place in the cell," says Mantas Gabrielaitis. "We found that the calcium-binding proteins in hair cells help to focus the calcium signal at the synapse, hereby allowing for a transmission of the auditory stimuli to the brain with minimum energy cost."
The Göttingen researchers Moser and Wolf point out that with the results of their basic research “We can now understand better how the key sensory cells of the hearing sense “provide maximum performance without wasting energy.”