
Developmental Biodynamics
Shoba Kapoor
1. Cilia driven flow of the Cerebrospinal fluid
The four ventricles of the mammalian brain are interconnected cavities that contain the cerebrospinal fluid (CSF). Along the walls of the ventricles, ependymal cells move the CSF via the whip-like movement of their motile cell protrusions called cilia. The direction of the cilia beating determines the near wall flow direction and is regulated by planar polarization of ependymal cells.
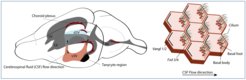
On the right is a scheme of ependymal cells with cilia bundles. Both position of the cilia bundle and rotation of each cilium within the bundles is polarized towards the flow direction.
In collaboration with Dr. Stefano Villa and his group ‘Dynamics of living matter’, we study the cilia driven flow of the cerebrospinal fluid in the ventral third ventricle (v3V) of the mammalian brain. We plan and execute experiments on brain explants or in vitro grown ependymal cells, while the group ‘Dynamics of living matter’ performs quantitative image analysis of the recovered data. This collaboration enables us to analyze the properties of the approximately 25.000 cells that decorate the wall of the v3V.
Our study started with the recording of the near wall bead movement that is caused by ciliary beating on v3V explants [1]. These recordings yield flow maps that show the v3V flow pattern and enable predictions of a possible cilia driven transport network in the brain.

Immunostaining and large-scale confocal microscopy experiments help correlate the flow directions from the above described bead tracking experiments and the polarity of individual ependymal cells. Because of large scale analysis methods, we describe flow-pattern and cell polarity correlation in the v3V of developing, adult, aging, and mutant mice. The algorithms to analyze cell and tissue wide polarity are provided by Dr. Christian Westendorf (University of Leipzig).
Immunostaining and large-scale confocal microscopy experiments help correlate the flow directions from the above described bead tracking experiments and the polarity of individual ependymal cells. Because of large scale analysis methods, we describe flow-pattern and cell polarity correlation in the v3V of developing, adult, aging, and mutant mice. The algorithms to analyze cell and tissue wide polarity are provided by Dr. Christian Westendorf (University of Leipzig).
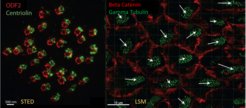
We also integrate DIC microscopy and fluorescent liposome imaging to record beating cilia bundles. We analyze these experiments to research the interplay between the observed flow pattern and the physical properties of the cilia beat within bundles.
The aim is the precise description of the flow network in order to understand its origin, development and it’s function.
In the near future, we plan to proceed in two ways. First, to generate an in vitro system that simulates the scenario of the v3V near wall flow. Secondly, to continue the research in freshly isolated v3V explants in wildtype and mutant mice, to discover genes that are possibly involved in the establishment of such a complex network.
[1] Faubel R., Westendorf, C., et al. (2016). Science, Vol 353,Issue6295,pp.176-178
2. 3D extrusion bioprinting of engineered human myocardium
Heart failure is a common, costly, and potentially fatal condition, in which the heart can’t pump enough blood to meet the body’s needs. In 2015 it affected about 40 million people globally [1]. Our collaborators Prof. Zimmermann et al. have shown that heart muscle implants can be generated in vitro [2]. These implants when attached surgically, are hoped to increase ejection fraction. In collaboration with the University Medical Center Göttingen and the MPI for Biophysical Chemistry, we are developing a multi-physics model for patient specific heart beating. Our group is interested in extruding microfibers that carry human fibroblasts and cardiomyocytes. This tissue will be further characterized for their mechanical and electrophysiological properties by our graduate student Sahar. We are thankful to Prof. Zimmermanns group, who will provide cardiomyocytes for this study.
In the future we would like to establish a cell culture of cardiac purkinje cells. We are interested in loading the above described microfibers with purkinje cells as they propagate electric signals faster than cardiomyocytes.
[1] Vos, T., Allen, C., Arora, M., et al. (2016). The Lancet, 388(10053), 1545–1602.
[2] Zimmermann, W.-H., Melnychenko, I., et al. (2006). Nature Medicine, 12(4), 452–458.