Biofluidics and Biomechanics
Yong Wang
Research interests
We are a group of physicists, biologists and engineers who are interested in interdisciplinary research especially biofluidics and biomechanics. In collaboration with other groups around the world, we are mainly investigating:
A. Failing heart regeneration

Heart failure is a common, costly, and potentially fatal condition in which the heart can’t pump enough blood to meet the body’s needs. In 2015 it affected about 40 million people globally [1]. Our collaborator Prof. Zimmermann et al. have shown that diseased matched heart muscle implants can be generated in vitro [2]. These implants when attached surgically are hoped to increase ejection fraction. In collaboration with the University Medical Center Göttingen and the MPI for Biophysical Chemistry we are developing a multiphysics model for patient specific heart beating. We are using medical imaging technologies for recovering the heart geometry, contraction pattern and mechanical properties. Since the patient-specific tissue properties and hence model parameters play a crucial role on heart function, we also investigate the mechanical properties of heart muscle both experimentally and numerically. The goal is to understand better heart pumping, find the optimal solution of the EHM configurations, and provide the EHM construction process with a personalized EHM patch design and its placement. Besides cardiac mechanics, we are also working on low-energy control of cardiac arrhythmia, turbulent blood flow within the heart and medical imaging.
The animation shows blood flow in the left part of a heart. The data was recorded with real-time MRI by Prof. M. Uecker.
B. Cilia coordinated flow
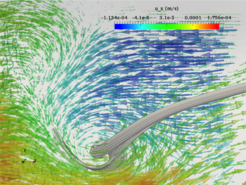
CSF conveys many physiologically important signaling factors through the ventricular cavities of the brain. The walls of the ventricular cavities are covered with motile cilia that were previously thought to generate a unidirectional CSF flow. A complex transport network driven by coordinated motile cilia inside the ventral third ventricle (v3V) of mammalian brain was recently reported [3]. A cilia-based switch that reliably alters the flow pattern so as to create a dynamic subdivision that controls substance distribution within the v3V was also observed experimentally. In collaboration with the MPI for Biophysical Chemistry and University of Pittsburgh, we now study the contribution of the ciliary network to overall CSF flow in wide type and mutant mice. We are also interested in other tails such Chlamydomonas flagella, cilia in the embryonic node and sperm.
The figure shows cilia induced separatrix and whirl in the v3V. The arrows and grey curves are local velocity vectors and streamlines respectively, obtained numerically.
C. Human upper airway

Common surgeries for correcting the human upper airway (UA) obstruction include septoplasty, tonsillectomy and adenoidectomy. In the United States, about 600,000 children undergo adenotonsillectomy operation each year. However, the success rate of these operations is only about 50% due to lack of information on where the flow is constricted and where the UA dynamically collapses. Thus, understanding the UA flow properties and accurately locating the obstruction are of practical importance. With the scientists at University of California, Irvine, we performed direct numerical simulations (DNS) with the state-of-the-art lattice Boltzmann method (LBM), and investigated the turbulent flows in patient-specific UAs reconstructed from CT and OCT scan data. A method based on the time-averaged first and second spatial derivatives of pressure was proposed to locate the region of the UA obstruction [4]. Moreover, together with other groups, we studied airflow in the UA of an OSA patient with several methods, including the PIV, DNS, LES and RANS, and compared those methods.
The animation shows fluid particles moving in the UA during inspiration. The flow was resolved by DNS with the LBM. The geometry was from Prof. J. Kimbell.
D. Blood flow and others

Together with other groups in Goettingen, we study the 4D blood flow inside human heart. With both experiment (MRI, CT) and numerical simulation (FSI), we recover the time-dependent blood flow and help the doctors to improve diagnosis, prevention and therapy for heart diseases. We also study the reduction of blood hemolysis in autotransfusion devices. Moreover, we are interested in flow stability [5], numerical methods, such as the LBM [6] and it's applications to compressible flow, multiphase flow and FSI.
The figure shows the blood pool in the left part of a heart, reconstructed from CT scan data. The CT data was from Prof. J. Lotz.
References:
[1] Vos, T., Allen, C., Arora, M., et al. (2016). The Lancet, 388(10053), 1545–1602.
[2] Zimmermann, W.-H., Melnychenko, I., Wasmeier, G., et al. (2006). Nature Medicine, 12(4), 452–458.
[3] Faubel, R., Westendorf, C., Bodenschatz, E., & Eichele, G. (2016). Science, 353(6295), 176–178.
[4] Wang, Y., & Elghobashi, S. (2014). Respiratory Physiology & Neurobiology, 193, 1–10.
[5] Bai, X.-D., Zhang, W., Guo, A.-X., & Wang, Y. (2016). Physics of Fluids, 28(4), 44102.
[6] Y L He, Y Wang, Q Li. Lattice Boltzmann method: Theory and applications. Beijing: Science Press, 2009.