Dynamics of Living Matter
Stefano Villa
Research interests
Cilia driven flow of the Cerebrospinal fluid
In collaboration with Dr.Shoba Kapoor and Dr.Yong, we study the cilia driven flow of the cerebrospinal fluid (CSF) in the ventral third ventricle of the mammalian brain. Ventral third ventricle (v3V) is a thin cavity located in the central part of the brain containing CSF. There, CSF flows following a complex pattern which results from the dynamics of ciliated cells covering the inside of the ventricle. The function of such a complex pattern remains largely unknow.
In particular, we analyze image data of murine brain explants and in-vitro cell cultures provided by the group Developmental Biodynamics.
We indagate how the flow pattern arises from the cilia motion by quantifying and correlating different dynamic and static properties of the tissues:
- cell polarity obtained from fluorescent microscopy of stained tissues
- cilia bundle dynamics accessed from DIC microscopy
- particle and fluorescent liposomes tracking in bright field microscopy
We are mainly interested in deepening the interplay between biochemical signaling and hydrodynamics during cell development in order to understand the pattern function and its formation process.
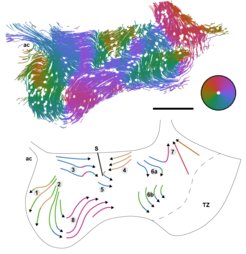
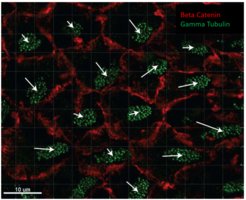
References
R. Faubel et al. ‘Cilia-based flow network in the brain ventricles’, Science 353.6295, 176-178 (2016)
Effect of in-plane shear on cell dynamics, structure and self-organization
Cells are extremely complex systems able to interact with the surrounding environment via biochemical, physical and mechanical stimuli. As a result of these interactions, they adapt their physical properties in complex feedback mechanisms. A close interplay exists therefore between mechanical stimuli and cells properties, that becomes particularly interesting when studying systems where presence of stresses is particularly determinant as for tissue differentiation, tumor metastasis progression and dynamics of tissues actively exerting stresses, like muscles and in particular heart.
In order to deepen the interplay between cell behavior and mechanical stimuli, we developed a device for the in-plane shear of cell monolayers. The device is designed to be easily mounted on commercial live cell imaging microscopes, in order to monitor with typical imaging methods (phase contrast, DIC, fluorescence, …) the evolution of the monolayer during the application of the shear for long timescales.
With this device we are currently conducting measurements on three different systems:
- Effect of in-plane shear on pattern formation in amoeba colonies
Dictyostelium discoideum (D.d.) is a peculiar amoeba that goes through different stages during its life. When nutrients became scarce, a population of D.d. starts an aggregation process forming first aggregates of about 105 cells, that then became a multicellular slug and subsequently a fruiting body. From the fruiting body, spores spread in the environment and unicellular individuals emerge, starting a new cycle.
They represent a straightforward example of pattern formation in excitable media, as the aggregation process is driven by chemotaxis through the formation of spirals propagating across the sample.
We are deepening the effect of external stresses on pattern formation because of the close interplay between mechanical action and tissue differentiation, documented in different living systems during their developmental phase.
We observed that the application of a periodic in-plane shear heavily affects the pattern formation, by altering spirals formation. We are currently deepening this observation by comparing with models and by tuning conditions such has sample geometry, shearing frequency and illumination.
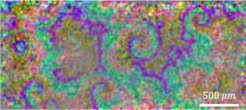
- Shear-induced dynamics of confluent cells monolayers
Cells are complex active elements that adapt their physical properties in complex feedback mechanisms when subjected to external biochemical, physical and mechanical stimuli. Confluent monolayers of cells constitute therefore an extremely complex active medium, whose response to external mechanical stimuli is still largely unexplored. A further comprehension of such response is of paramount interest for understanding the tissue differentiation at the early stage of embryonic development, when stresses contribute to tissue organization.
We are experimentally investigating the dynamics and shape of epithelial cells subject to an external in-plane shear deformation, correlating the applied step shear deformation with the affine and non-affine dynamics of the cells. We compare the experimental observations with the output of numerical simulations with Vertex model.
- Experimental investigation of heart muscle fibers self-organization
Heart pumping efficiency is granted by the contraction of Cardiomyocytes (CM): contractile cells organized in fibers and surrounded by extracellular matrix (ECM). The latter is a network of macromolecules and minerals that provide structural support to the cells. The mesoscopic contraction of the systems takes place along the fibers. In vitro experiments revealed that a mixture of ECM and CM spontaneously organize in fibers. We are studying the dynamics of CM in vitro to characterize the dynamics of fiber formation. The working hypothesis is that mechanical stresses propagating within the tissue drives the dynamic of CM to minimize stresses on the cells.
Collective dynamics and phase transitions in cell monolayers
Work in collaboration with University of Milan and IFOM institute on the characterization of confluent cell monolayers undergoing a jamming phase transition, characterized by the rising of collective migration (Mov.2.1) and a transition from a solid-like to a fluid-like state. Flocking cell monolayers are interesting models for the study of phenotypes associated to cancer evolution towards metastasis.
In particular, we currently study the shape deformations cells nuclei undergo in jammed and flocking monolayers (Mov.2.2) correlating them with the local dynamic state of the tissue (Fig.2.1) .

References
E. Frittoli, A. Palamidessi et al. ‘Tissue fluidification promotes a cGAS–STING cytosolic DNA response in invasive breast cancer.’ Nat. Mat., 22.5, 644-655 (2023).
S. Villa et al. ‘Non-invasive measurement of nuclear relative stiffness from quantitative analysis of microscopy data.’, Eur. Phys. J. E, 45.5, 1-18 (2022)
R. Cerbino et al. ‘Disentangling collective motion and local rearrangements in 2D and 3D cell assemblies.’ Soft Matter 17.13, 3550-3559 (2021).
Rheomicroscopy of soft materials
Work in collaboration with University of Milan on the characterization of mechanical properties of viscoelastic soft materials. We work on the development and characterization of a microscope adapted stress controlled shear cell, assembled at the Biometra department of University of Milan. Sample strain associated to an imposed stress is assessed trough an optical measurement, thus enabling the recovering of mean mechanical properties of the sample. The simultaneous imaging of the sample allows to correlate macrorheological properties with local dynamics of the sample at the micrometric scale, thus opening the way to a deeper comprehension of the microscale structural origin of macroscopic mechanical properties of soft materials.
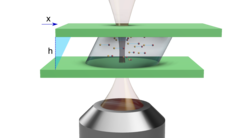
References
S. Villa, P. Edera et al. ‘Quantitative rheo-microscopy of soft matter’, Frontiers in Physics, 905 (2022).
Dynamics of colloids in the vicinity of gas-liquid interface
Project in collaboration with University of Montpellier on the description of the dynamics of micrometric particles of different shapes in the vicinity of air-water interface. Measurements are made with a Dual Wave Reflection Interference Microscopy – developed at Charles Coulomb laboratory (Montpellier) - enabling 3D tracking of colloidal dynamics. The study is devoted to the understanding of the physics underlying the dynamics of bacteria and biofilms in the vicinity of water surface and, in the waste water treatment framework, the approach and trapping of micrometric pollutants at the surface of water.
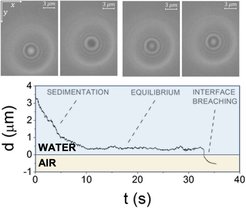
References
S. Villa et al. ‘Dynamics of prolate spheroids in the vicinity of an air–water interface.’ Soft Matter, 19 14, 2646-2653 (2023).
S. Villa et al. ‘Microparticle Brownian motion near an air-water interface governed by direction-dependent boundary conditions.’ J. Coll. Int. Sci., 629, 917-927 (2023).
S. Villa et al. ‘Motion of micro-and nano-particles interacting with a fluid interface.’ Adv. Coll. Int. Sci., 284, 102262 (2020).
S. Villa et al. ‘Multistable interaction between a spherical Brownian particle and an air–water interface.’ Soft Matter, 16 4, 960-969 (2020).