Synthetic Active Systems
Isabella Guido
Synthetic cilia
Cilia are microscopic hair-like structures that present a rhythmic waving or beating motion and are found on the surface of almost all mammalian cells and on the body of some protozoan organisms. They are used for fluid flow based transport (e.g. removal of pollutants in the trachea) or for the locomotion in viscous fluid environments. In our work we aim to develop synthetic ciliated systems able to propel themselves or to move fluids across a fixed surface. For this purpose we employ a bottom-up approach for assembling a simple system made of few building blocks adapted from natural cilia, namely microtubules and motor proteins. Using Kinesin-1, a processive motor powered by ATP hydrolysis, we synthesized a system containing MT bundles that are free to move in all planes, deviating from the conventional gliding assay. By binding them to a surface using a suitable anchor system, we are able to observe the microtubules-motor protein system oscillations in a manner that closely mimics ciliary movement. The issue that we are addressing in our experiments is: how simple is the simplest system that is able to beat?
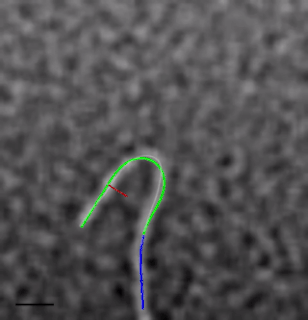
Active Networks
Networks of biofilaments and motor proteins are important model systems for the understanding of the out-of-equilibrium behaviour of the cellular cytoskeleton. In nature, they are involved in key processes in cells such as migration, division, mechanosensing and cytoplasmic transport. Designing in vitro setup of such systems can help their characterization thanks to the fewer components used and the possibility to introduce controlled perturbations. In my group we assemble such network by using polymerized microtubule, multi-headed kinesin-1 motor proteins, poly(ethylene glycol) or PEG and ATP. The non-equilibrium nature of the system is due to kinesin-1 that in the presence of ATP moves along the microtubules. By adding the non-adsorbing polymer PEG attractive interactions between microtubules are induced through depletion force that leads to bundle formation. By confining the network into a defined geometry, we observe the formation of instabilities resulting from the contraction and the extension the network is subjected to. These results clearly show how microscopic interactions between biopolymers and motor proteins give rise to collective dynamics at a different scale.
Electrotaxis in Dictyostelium discoideum
In my group we are also slightly interested in the mechanics of cells under the stimulus of a DC electric field and on the dynamics of intracellular molecular signals that are triggered by the electrical stimulation. Cells show a sensing mechanism for the applied electric stimulation, which enable them to get polarized and migrate toward the cathode. A fascinating question is understanding the molecular signals underlying this mechanism. Dictyostelium discoideumis an excellent model system to study the molecular mechanisms of cell migration and polarity signals in the electric field and this analysis may provide a complementary insight into the dynamics of subcellular and molecular processes underlying cell movements.
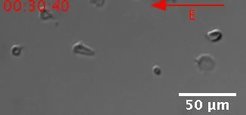
Video © 2020 Guido et al, available here