Particles in Turbulence
Jan Moláček
Droplet dynamics in clouds

Although everyone has come into contact with a raindrop, few of us are aware of the plethora of physical processes and complex interactions that are involved in creating one. Its origins in the cloud of droplets created by condensation on aerosol nuclei suspended in the air are relatively well understood, although even here a few surprises still keep cropping up [1]. Similarly well known are the last stages of a raindrop as it falls towards the ground and gains size by coalescing with smaller droplets in its path. The mystery lies in the transition between the two stages as the time it takes for the first few collisions of a drop to happen has proven difficult to predict using simple models. It is now believed that turbulence plays a major role in speeding up this process [2] by preferentially distributing the cloud droplets in space and enhancing their collision rates by creation of high-acceleration events which "detach" droplets from their local flow velocity [3]. To complement our novel laboratory experiments [3,4] in this area, we have built a field setup at the environmental research station Schneefernerhaus , situated near Germany's highest mountain Zugspitze. Using a sophisticated particle-tracking setup, we obtain simultaneously the spatial distribution of cloud droplets with high spatial resolution, and the relative inter-droplet velocities with high temporal resolution. These data allow us to directly and indirectly measure the collision rate of cloud droplets. Combined with simultaneous measurements of the underlying turbulence properties and information about the droplet size, we hope to improve the existing parametrisation of the collision rate as a function of the turbulence dissipation rate and droplet Stokes number. Currently we are investigating various methods to measure individual droplet diameters to improve the versatility of our results.
Droplet collisions
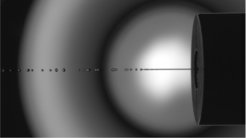
Knowing the collision rate of cloud droplets goes a long way towards being able to predict the evolution of drop size distribution with time and thus predicting the onset of rain, but it would not be complete without a grasp of the coalescence efficiency. This quantity describes the fraction of droplets that coalesce after a successful collision. Due to its dependence on many parameters, such as the drop relative velocity, surface tension, density, rotation rate, electric charge, purity of the surface and density of the surrounding gas, its value is still a matter of active research for many parameter regimes. We aim to investigate the effects of a few select parameters on the coalescence efficiency by colliding pairs of droplets in a controlled laboratory environment. A large part of the experimental challenge is the creation of a drop with the desired characteristics. Using our high-precision 3D-printer from NanoScribe GmbH, we are able to experiment with various three-dimensional designs of nozzles for droplet generation, which would not be possible to manufacture by more traditional methods.
Droplet impact on liquid and solid surfaces

The original motivation for our theoretical and experimental investigation of droplet interactions with liquid and solid surfaces was to understand and predict the behaviour of the droplets bouncing on a vertically vibrated liquid bath. In some parameter regimes, such droplets become horizontally unstable and begin to "walk" - move across the surface, propelled by the interaction with the wavefield they create on the liquid surface with each bounce. The various modes of vertical bouncing combined with the richness of the drop-wave and wave-boundary interaction produce many interesting phenomena previously thought to be confined to the realms of quantum mechanics [5]. Being able to accurately model and predict the complex vertical and horizontal dynamics of a walking droplet allows one to more quickly explore various scenarios and guide the experiments in interesting directions. To model such a complex system, one needs to start from the fundamentals - if a droplet hits a liquid surface with a given velocity, given rotation rate and at a given angle, what will those quantities be after the droplet rebounds ? This problem has been studied phenomenologically (especially for impact on solid surfaces), but quantitative studies, especially in the low- to mid-Weber number regime, have been lacking. We are striving to obtain the basic experimental data describing the drop-surface interactions and to develop simple yet accurate theoretical models that can reproduce it.
Lagrangian particle tracking
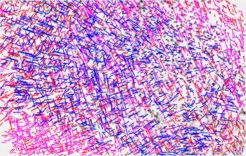
Lagrangian particle tracking (LPT) is a popular method of investigating the properties of a flow by means of tracking over time the movement of small, near-tracer particles suspended in it. This method has gained in popularity in recent years over the more traditional particle tracking velocimetry (PTV) thanks to advances in the tracking algorithms, such as the "Shake-the-Box" algorithm [6], which have substantially increased the numbers of particles that are possible to track simultaneously. The drawbacks of this method lie in the fact that it relies on multiple high-speed cameras (typically four for dense seeding) and a strong source of illumination (required since the particles are typically ~10um in size and need to be imaged with very short exposure times), which implies high capital cost. We are developing algorithms that allow reliable LPT with smaller number of cameras (two to three) and a weaker illumination source.
The LPT method has been developed to study the properties of the flow, for which only the tractable seeding density (higher seeding density means better spatial resolution of the flow) and the fraction of ghost particles (a measure of the method's accuracy) are important. However, for the purposes of studying the properties of particles in turbulence (such as droplets in clouds), the focus is on the particles themselves. This can lead to unforeseen biases in the collective statistics, such as the measured radial distribution function, due to the differential likelihood of observing a particle in a more or less densely populated region of space. We are investigating ways to detect and combat the LPT biases in such scenarios.
References
- [1] J. Svensmark, et al., J. Geophys. Res. Space Physics, 121, 8152-8181 (2016)
- [2] A. Pumir, et al., Annu. Rev. Condens. Matter Phys., 7, 141-70 (2016)
- [3] G. P. Bewley, E.-W. Saw, E. Bodenschatz, New J Phys., 15, 083051 (2013)
- [4] E.-W. Saw, G. P. Bewley, et al., Phys. Fluids, 26, 111702 (2014)
- [5] J.W.M. Bush, Annu Rev. Fluid Mech., 47, 269-292 (2015)
- [6] D. Schanz, S. Gesemann, A. Schroeder, Exp. Fluids, 57 (2016)